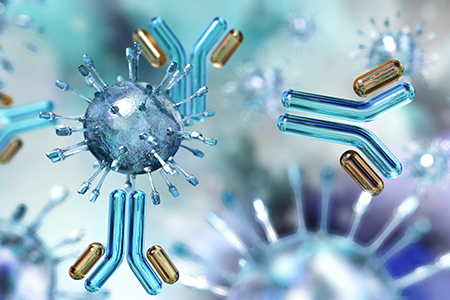
Syngeneic Mouse Models Demonstrate Utility in Immuno-Oncology Research
A syngeneic tumor model combines a complete, functioning immune system with an engrafted tumor derived from a genetically identical mouse strain. With a genetic match between tumor and host, tumor engraftment is successful in these models.Because they enable the study of tumor-immune system interactions in response to treatment, syngeneic models are increasingly the research tool of choice for many investigators conducting immuno-oncology studies. To date, over 100 murine tumor cell lines have been developed from inbred mouse strains for use in syngeneic studies (though this number is still eclipsed by the much larger number of human cancer cell lines available for xenograft studies).
Syngeneic models offer critical advantages for immuno-oncology drug discovery:
- A genetic match between tumor and host enables studies on the interaction between tumor cells, immune cells, the associated stroma, and humoral effects.
- Compatibility with both existing and customized genetically engineered models (GEMs) generated on defined backgrounds, providing investigators with flexibility in conducting mechanistic and translational studies.
- Ability to explore the microbiome's role in disease development and treatment, enabling researchers to use germ-free mice or mice associated with defined microbiota to study how the interaction of the host and its microbiome impact cancer growth and response to therapeutics.
- The hosts on which syngeneic models are based are relatively inexpensive and available in large quantities.
For effective preclinical immuno-oncology modeling, it is necessary to use a tumor-bearing host with the immune functionality needed for a particular therapeutic modality. Both syngeneic mouse models and humanized immune system (HIS) models fit this requirement; however, due to their inherent differences, each is more appropriate than the other for certain types of studies. For example, a syngeneic model using a GEM that expresses a human drug target can be used to study non-immune cells/tissues that express the humanized gene of interest, enabling researchers to gain mechanistic insights and perform off-target analyses. On the other hand, HIS models are not compatible with GEMs for use in mechanistic studies.
Factors to Consider in Selecting a Syngeneic Model for Immuno-Oncology Applications
When selecting a syngeneic mouse model for preclinical immuno-oncology research, several considerations should be taken into account.Genetic Background
Engrafting a syngeneic tumor without the risk of rejection requires a close genetic match between the tumor and host at all major and minor histocompatibility loci. The wide range of inbred mouse strains with defined, consistent genetics makes this degree of genetic matching possible. However, of the hundreds of inbred strains available, a relatively small number are routinely used in syngeneic studies, with the C57BL/6 and BALB/c representing the most-often used inbred strains for these research applications. Mouse model providers like Taconic Biosciences maintain rigorous control of the genetic quality of their inbred mouse colonies to ensure consistency of genetic background and to prevent genetic drift.In addition to strain background, substrain should be considered when selecting a host for a syngeneic study. Substrains generally are histocompatible and will accept tumors from other members of the same parental strain. For example, tumors from a C57BL/6J mouse should successfully engraft into a C57BL/6NTac mouse. However, it is important to consider that substrains may perform differently in various applications.
When conducting syngeneic studies in a GEM, it is vital to validate the model's genetic background. This is easily done using single nucleotide polymorphism (SNP)-based assays. Results from SNP testing may be used to calculate the percentage of genetic contamination from alternate strains, providing insights as to whether it is best to backcross the GEM to a preferable background. In such cases, successive backcrossing of animals carrying the genetic mutation to a defined inbred strain is a commonly used approach. After approximately ten backcross generations, the resulting strain should be 99.9% identical to the recipient inbred strain, except for a small region flanking the genetic modification. This technique is highly effective; however, it takes about three years, which can greatly slow a study's progress. Utilizing genome-based markers makes it possible to generate congenic strains in about half that time through accelerated backcrossing3.
Health Status
Health status is always an important factor when choosing a mouse model for preclinical research. Animals at the specific pathogen-free (SPF) and specific and opportunist pathogen-free (SOPF) health profiles are widely available and acceptable at most research facilities, though care must be taken when importing SPF animals into a facility that houses immunocompromised models.Two considerations should be kept in mind regarding the SPF and SOPF health statuses:
- These health statuses are exclusionary; they define excluded pathogens and/or opportunistic agents, but typically do not address the presence or absence of any specific commensal microorganism.
- Definitions of the terms "SPF" and "SOPF", as well as the specific pathogens, opportunists, and commensal organisms they exclude, may vary across vendors and research facilities. The use of strict bioexclusion practices is required to maintain animals of different health profiles within the same facility, while global harmonization of health standards helps to ensure consistency across vendors or facilities.
Microbiome
The host microbiome has the potential to impact how a tumor grows, how it responds to a therapeutic, and its translatability to human tumors. Many factors can have a profound influence on the microbiome of a syngeneic host—including its health status, the processes used at its production site, transportation methods, and other practices of the vendor providing the host, as well as the husbandry and biosecurity processes and practices in use at the research site4.The microbiome's impact on the efficacy of a therapeutic has been well documented, particularly with regard to immune checkpoint inhibitors such as anti-PD-1/PD-L1 and anti-CTLA-4 treatments. Specifically, Bifidobacterium and Bacteroides fragilis have been shown to regulate tumor growth and response to therapy. In one study, following oral administration of B. fragilis and treatment with the CTLA-4-specific 9D9 mAb (a mouse surrogate for the immune checkpoint inhibitor ipilimumab), investigators observed remission of mouse sarcomas5.
Though they are highly effective in a subset of cancer patients, checkpoint inhibitors have proven not at all effective in others. Researchers have conducted many studies to test the hypothesis that the variation in checkpoint inhibitor efficacy may be related to variations in patients' microbial compositions. Three landmark studies published in Science in 2018 provided evidence of a correlation between certain microbial strains and a positive response to immune checkpoint inhibitor treatment. Investigators found that a greater abundance of Akkermansia muciniphila, Faecalibacterium, and other specific commensals in the gut microbiome was correlated with positive patient response to checkpoint inhibitor therapy6-8. Germ-free mice colonized with microbiota from patients who responded effectively to the therapy and were administered the same therapeutic experienced reduced tumor growth.
Since the microbiome can affect a syngeneic model's performance and the reproducibility of study results, measures should be taken to maintain microbiome consistency within and across syngeneic studies. In particular, variables such as the source of the mice, sex, stress, type and sterility of diet, water source (e.g., reverse osmosis, autoclaved, acidified, or chlorinated), bioexclusion practices, type and sterility of bedding material, cage density, and cage change frequency all should be controlled to the degree possible. When conducting microbiome-related studies, the use of custom microbiome associations also can help improve consistency and reproducibility.
As immunotherapy continues to be the chief focus of cancer drug discovery, syngeneic models will continue to be a workhorse of the oncology research field. By providing a relevant model for tumor studies with the benefit of a complete, functioning immune system, syngeneic models offer investigators a very compelling option as they seek the most relevant preclinical model to advance their immuno-oncology research.