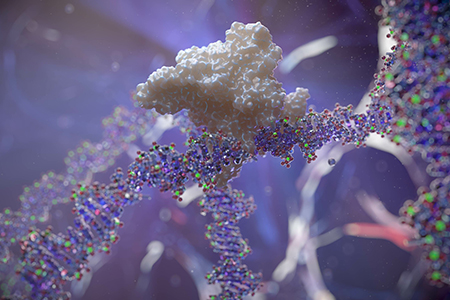
Applications of the Cre/LoxP System
The applications of Cre/loxP technology continue to grow. In a fundamental sense, this reflects the simple premise — a rearrangement of DNA sequences — and is only limited by the content or identity of those sequences. Yet while many complex applications exist in both the generation and use of mouse models, several basic and well-established uses remain quite powerful.Global or Ubiquitous Recombination
New mouse models generated through the use of homologous recombination (HR) in embryonic stem cells (ESCs) often contain one or more selection cassettes that are designed for removal. This is often facilitated by the presense of two flanking loxP sites inserted in the same orientation (this concept leads to the terminology of a "floxed" allele). Such removal simply requires one-time exposure to Cre recombinase. To ensure that the recombination is complete and universal, certain Cre models are designed to express Cre recombinase in a ubiquitous and constitutive fashion. Following a simple cross of one mouse harboring the floxed sequence to another harboring Cre, it is possible to obtain offspring which harbor both. Due to this Cre exposure, and after succesful recombination, such offspring will no longer possess the original targeted allele, but rather one in which the selection cassette has been excised. This is true in the germline as well and as a result, any offspring produced from further mating will inherit this recombined allele without the selection cassette, irrespective of their own inheritance of Cre. The recombined allele may then be propagated normally and it is no longer necessary to select for Cre inheritance in future generations. In contrast, it is recommended that Cre be selected against and actively removed from the breeding colony. This same approach can also allow for the generation of a constitutive knockout model, following a similar cross of any conditional knockout (cKO) allele to a Cre model that expresses Cre recombinase in a ubiquitous fashion.Conditional or Restricted Recombination
In contrast, it is often desirable to limit the extent of Cre-mediated recombination in a spatial and/or temporal fashion. A classic example arises when complete loss-of-function for a particular gene product results in lethality during development, but the researcher is concerned only with the study or function of that gene product in the adult animal. Due to the commonplace nature of this example, a vast multitude of Cre "driver" models have been created. Typically, these "driver" models limit the expression of Cre recombinase by placing it under the control of a promoter or enhancer sequence known to confer tissue- or cell-specificity. It is also common to limit the activity (though not the expression) of Cre recombinase temporally by utilizing one of several engineered versions of the enzyme which are fused to a ligand-binding domain of a human progesterone (e.g., CrePR) or estrogen (e.g., CreER) receptor, and thus regulated by exposure to synthetic ligands such as RU486 or tamoxifen, respectively. One alternative approach has also placed Cre recombinase expression under the control of a TetR-based system. Whether utilizing one or both of these strategies (spatial or temporal restriction), new Cre drivers are often first tested and validated through a cross with a "reporter" model. Reporter models often express a fluorescent protein only after exposure to Cre recombinase and removal of an upstream lox-STOP-lox cassette to allow for a convenient readout of activity. Though this strategy provides a first-pass understanding of Cre activity, differences in the extent of recombination are often observed when using different floxed alleles. Ideally then, a pilot study should be conducted for each unique combination of Cre driver and floxed allele studied, with the extent of recombination validated or confirmed directly in the tissue(s) or cell(s) of interest (e.g., through the examination of transcript levels or protein expression).To utilize such Cre drivers, a fairly simple approach may be used. First, performing a cross between one mouse harboring a floxed allele and another harboring Cre will generate offspring which carry a single copy of each. In certain situations, this may be sufficient to allow experimental use, though, in order to generate animals homozygous for the floxed allele (for example, to allow complete conditional knockout of a gene), a second cross is necessary. This can be a simple cross of the animal carrying one copy of each allele to a mouse harboring only the floxed allele. This will generate offspring homozygous for the floxed allele and either heterozygous or wild-type for Cre (among other genotypes). Apart from the example of a cKO model, floxed or conditional alleles are often generated for other purposes, such as conditional expression of a toxin or other ectopic protein.
Though it is common to utilize Cre-negative littermates as controls in such experiments, analysis of Cre-positive control littermates may also be beneficial. First, analyzing Cre-positive control mice can inform whether or not the Cre allele generates an adverse effect (e.g., haploinsufficiency, for knockout/knock-in alleles, disruption of an endogenous gene for randomly inserted transgenes). Second, Cre-positive offspring can act as controls for the ectopic expression of the Cre protein, as it can be toxic in different contexts or experiments. And finally, this analysis can ensure that the function of the Cre protein itself (and any unknown or undetected activity) does not have an impact on the phenotype of interest.
For the inducible forms of Cre based on fusion to a modified human estrogen receptor (e.g., CreER), tamoxifen is most often used to trigger recombination and can be delivered via multiple routes (e.g., subcutaneous injection, oral gavage, and/or diet administration). Often, the dosage is titrated to achieve the desired result: lowered to achieve mosaic recombination or increased (and/or administered multiple times) to ensure near-complete recombination. Appropriate tamoxifen administration protocols should ideally be determined empirically before use and performed identically on both experimental and control animals.
Ligand-Independent Recombination (Inducible Cre Drivers)
With inducible Cre drivers, Cre is sequestered in the cytoplasm in order to restrict its recombinase activity and is only thought to enter the nucleus after induction. However, ligand-independent Cre activity may occur at a low level and is likely due to proteolysis of the Cre fusion protein and subsequent nuclear transport. For example, our experience shows that crossing a ubiquitously expressed and inducible CreERT2 driver (Gt(ROSA)26Sor<tm9(Cre/ESR1)Arte>) to various floxed alleles and monitoring for recombination in the absence of tamoxifen treatment results in approximately 25% of those alleles displaying some degree of ligand-independent Cre recombination. Among those floxed alleles affected, the percent of animals examined containing one or more copies of the recombined allele varied from below 1% to as high as 98% (Table 1). This large variation is likely due to the confluence of multiple factors, including chromatin environment, expression of the target gene, and distance between loxP sites. However, within this dataset no clear correlation existed between this type of uninduced recombination and any single factor.Table 1 - Ligand Independent Recombination Observed with Exposure to ROSA CreERT2
Target Gene | Percent Recombination |
Ctla4 | 1.10% |
Ctsk | 21.50% |
Gpr17 | 11.50% |
Grn | 87.70% |
Havcr2 | 3.4% |
Insr | 0.10% |
Irf5 | 0.90% |
Lag3 | 35.60% |
Mrc1 | 0.50% |
Nlrp3 | 0.80% |
Nnmt | 0.90% |
Nod2 | 7.70% |
Otc | 35.50% |
Prmt5 | 1.00% |
Scn9a | 33.20% |
Tigit | 0.40% |
Txnip | 27.60% |
Txnip | 97.70% |
When pursuing breeding plans that aim to generate experimental animals with limited or restricted recombination, it is also important to be aware that recombination may still occur in unintended times and places. In severe cases, and if undetected, this may even introduce a risk of losing the floxed allele (please see the sections below). The best defense against this is to always keep a separate breeding colony for maintenance of the floxed allele, apart from experimental matings and never exposed to Cre. Doing so will eliminate the risk of the complete loss of the floxed allele.
Unintended Recombination
Cre drivers often show some level of expression in the germline or early embryo, whether or not this may be predictable. When this occurs, and one or more floxed alleles are also present or transmitted within the same cell, recombination may result2. Such recombination is often not desired and may therefore be referred to as unintended. If this occurs in either the male or female germline, then a recombined allele (△) may be inherited rather than a floxed allele. Without other complications, the animal which results may then be a compound heterozygote, containing one copy of the recombined allele and one copy of the floxed allele (△/fl) in all cells of the adult (with the intact floxed allele inherited from the parent in which germline recombination did not occur). If this instead occurs in the early embryo, then based on the exact timing and extent of recombination, the animal which results may be homozygous for the recombined allele (△/△), a compound heterozygote (△/fl), or mosaic (i.e., containing cells of two or more distinct genotypes). It is important to realize that in either case, unintended recombination (as detected in offspring) may actually occur independently from the inheritance of Cre. In the germline, this may occur due to recombination at the diploid stage of gametogenesis and the subsequent segregation of the Cre and recombined allele. In the early embryo, this may occur due to maternal expression of Cre in oocytes of a Cre-positive dam. Interestingly, this last phenomenon is a known characteristic of certain Cre drivers that can be taken advantage of in order to improve the efficiency of certain breeding plans (not described here).A growing awareness of the possibility for such issues has led to the publication of multiple articles detailing observations of germline recombination for specific Cre drivers, including those referenced in the table below (Table 2). Observations included below for specific Cre drivers without such a reference are based solely on our observations and experience at Taconic Biosciences. In many cases, this information can be used to help facilitate a decision as to the most appropriate mode of Cre transmission (i.e., paternal or maternal).
Table 2 - Germline Recombination and Recommended Transmission Strategy for Select Cre Drivers
CreDriver | Unintended recombination | ||||
---|---|---|---|---|---|
Formal Nomenclature | Common Name | Paternal | Maternal | Recommended Transmission | Reference |
Amhr2tm3(cre)Bhr | Amhr2-cre | - | Y | Maternal | |
Chattm2(cre)Lowi | Chat-cre | N | N | Both | 3 |
Ctsktm1(cre)Ska | Ctsk-cre | Y | Y | Paternal | 9 |
Cx3cr1tm1.1(cre)Jung | Cx3cr1-cre | Y | Y | Maternal | |
Emx1tm1(cre)Krj | Emx1-cre | Y | Y | Paternal | |
En1tm2(cre)Wrst | En1-cre | Y | Y | Both | 3 |
Foxg1tm1(cre)Skm | Foxg1-cre | Y | - | Both | 3 |
Foxn1tm3(cre)Nrm | Foxn1-cre | Y | N | Maternal | 7 |
Foxp3tm9(EGFP/cre/ERT2)Ayr | Foxp3-CreERT2 | N | N | Paternal | |
Grik4tm1(cre)Ksak | Grik4-cre | Y | Y | Both | 3 |
Lyz2tm1(cre)Ifo | Lyz2-cre | N | Y | Paternal | |
Lyz2tm1(cre/ERT2)Grtn | Lyz2-creERT2 | N | N | Paternal | |
Pvalbtm1(cre)Arbr | Pvalb-IRES-cre | Y | Y | Maternal | 5 |
Pvalbtm1.1(cre)Aibs | Pvalb-2A-cre | Y | Y | Maternal | 5 |
Rgs9tm1(cre)Yql | Rgs9-cre | Y | Y | Paternal | 8 |
Rorbtm1.1(cre)Hze | Rorb-cre | N | N | Both | 3 |
Slc17a6tm2(cre)lowl | Glut2-cre | N | N | Both | 3 |
Sox17tm1(icre)Heli | Sox17-cre | N | N | Maternal | |
Ssttm2.1(cre)Zjh | Sst-cre | N | N | Both | 3 |
Tg(Adora2a-Cre)KG139GSat | A2a-cre | N | N | Paternal | 3 |
Tg(Alb-cre)21Mgn | Alb-cre | N | N | Both | |
Tg(Camk2a-Cre)159Kln | Camk2a-cre | Y | Y | Maternal | |
Tg(Cd8a-cre)1Itan | Cd8a-cre | N | N | Both | |
Tg(Cdh16-Cre)91Igr | Cdh16-Cre | N | N | Both | |
Tg(Drd2-cre)ER44Gsat | Drd2-cre | N | N | Both | |
Tg(GFAP-cre)25Mes | GFAP-cre | Y | Y | Paternal | 4 |
Tg(Gfap-cre)77.6Mvs/2J | GFAP-cre | Y | N | Maternal | 3 |
Tg(Gpr26-cre)KO250Gsat | Gpr26-cre | Y | N | Maternal | 3 |
Tg(Grik4-cre)G32-4Stl | Grik4-cre | N | N | Both | 3 |
Tg(KRT14-cre)8Brn | Krt14-cre | Y | Y | Both | |
Tg(Lck-cre)1Cwi | Lck-cre | Y | Y | Maternal | |
Tg(Lck-cre)548Jxm | Lck-cre | N | N | Both | |
Tg(Mx1-cre)1Cgn | Mx1-cre | - | - | Paternal | |
Tg(Nes-cre)1Kln | Nes-cre | Y | Y | Paternal | 4 |
Tg(Pcp2-cre)2Mpin | Pcp2-cre | Y | N | Maternal | 3 |
Tg(Pdx1-cre)6Tuv | Pdx1-cre | Y | Y | Paternal | |
Tg(Plp1-cre/ERT)3Pop | Plp1-creERT2 | N | N | Paternal | |
Tg(Scnn1a-cre)2Aibs | Scnn1a-cre | N | N | Both | 3 |
Tg(Six3-cre)69Frty | Six3-cre | Y | Y | Both | 3 |
Tg(Sox10-cre)1Wdr | Sox10-cre | Y | Y | Maternal | |
Tg(Syn1-cre)671Jxm | Synapsin1-cre | Y | Y | Maternal | |
Tg(Thy1-cre/ERT2,-EYFP)HGfng | Thy1-creERT2 | N | N | Paternal | |
Tg(Vil1-cre)997Gum | Vil1-cre | N | - | Both | |
Tg(Vil1-cre/ERT2)23Syr | Vil1-creERT2 | N | N | Paternal | |
Tg(Wnt1-cre)2Sor | Wnt1-cre | Y | Y | Both | |
Twist2tm1(cre)Dor | Twist2-cre | Y | N | Maternal | 6 |
Viptm1(cre)Zjh | Vip-cre | N | N | Both | 3 |
Legend for Unintended Recombination Columns
No data available | - |
Data available, no recombination observed | N |
Data available, recombination observed | Y |
If no prior experience is available to inform the decision, then the preferred direction of transmission can also be determined empirically. To do so, an animal of either sex that harbors both the Cre and the floxed alleles may be mated to a wild-type counterpart. Offspring may then be screened for the floxed and recombined alleles (regardless of their Cre inheritance) and the direction of transmission that minimizes any unintended recombination would then be preferred. Again, these pilot studies should always be performed with the exact Cre driver and floxed allele of interest if possible, as recombination rates will typically vary for each specific combination.
Notably, such a pilot study only speaks to potential germline recombination if the analysis is limited to offspring that do not inherit Cre. Unintended recombination which occurs in offspring that inherit both Cre and the floxed may occur due to activity in either the germline or early embryo. To test for unintended recombination in the early embryo alone, a cross between one animal harboring Cre (alone) and another harboring the floxed allele (alone) may be performed, with recombination then assessed in offspring that inherit both. From the same type of cross, examination of Cre-negative offspring born to a Cre-positive dam may be used to assess recombination due to any maternal expression of Cre (i.e., deposition in the oocyte).
If recombination is detected unexpectedly during routine genotyping (e.g., in a tissue such as an ear or tail biopsy), unintended recombination in the germline or early embryo may be responsible (as discussed above). Importantly however, recombination can also occur locally within the tissue biopsy taken, if the animal in question has also inherited the Cre allele. Just as with recombination that may occur in the germline or early embryo, such recombination in adult tissues taken for biopsy may be unexpected. Therefore, both may offer a potential explanation for the same finding. Crucially, though, it is not possible to completely rule out either using PCR genotyping alone. Either event may occur in specific situation or instance, and the two are not mutually exclusive.
Detection and Prevention
As a best practice, all animals should be screened for both the floxed and recombined (△) alleles at each generation to help detect unintended recombination. Further, both assays should first be validated with positive and negative controls. When working with a new floxed allele, a positive control for the recombined allele may need to be generated in vitro or through intentional creation in vivo (e.g., use of a Cre driver with ubiquitous expression). Following this practice can lead to the detection of the floxed allele alone (no recombination), the recombined allele alone (full recombination), or both (partial recombination).If full or partial recombination is detected in animals that have not inherited Cre, these animals should not be used if possible. Further, this clear evidence of unintended recombination in the germline or early embryo may warrant changing the direction of Cre transmission (e.g., maternal to paternal) in order to minimize the production of such genotypes — especially if this issue was not originally considered or examined at the outset.
If partial recombination is detected in animals that have inherited Cre, these animals should only be used with a full understanding of the implications. Notably, that unintended recombination often cannot be discounted. In a best-case scenario, local recombination alone (e.g., within an ear biopsy used for genotyping purposes) may be responsible. This may be expected, and based on known expression patterns of the promoter driving Cre expression, or unexpected. In a worst-case scenario, the animal in question may be a compound heterozygote, harboring one recombined allele and one floxed allele in all somatic cells and gametes. When using a cKO model, this may mean the animal is effectively heterozygous for the constitutive knockout. Further breeding of such an animal will lead to the propagation of that recombined allele. If two such animals are bred together, it may lead to the generation of offspring homozygous for the recombined allele (e.g., constitutive knockout). If such occurrences go undetected, there may be a risk for permanent loss of the floxed allele. Therefore, as a best practice and at each step in the breeding plan, one of the two breeder animals should always be sourced from a feeder colony maintained in the absence of Cre.
To eliminate concerns regarding potential germline recombination entirely, it is possible to utilize a breeding plan in which Cre is transmitted with the recombined allele by design. With this approach, experimental (fl/△) and control (fl/+) littermates may be generated simultaneously, and be homozygous (△/△) or heterozygous (△/+) (respectively) for the recombined allele (△) upon Cre exposure. If controls heterozygous for deletion of the gene-of-interest are not preferred, however, ensuring the breeder transmitting Cre is heterozygous for the Cre allele will also ensure the generation of both Cre-positive and Cre-negative fl/+ offspring, with the latter assumed effectively wild-type at the locus of interest.
Table 3 - Genotypes Generated from a Cross in Which Cre is Transmitted With a Recombined Allele Rather than a Floxed Allele (Cre/+; △/+ and +/+; fl/fl Breeders).
Genotype | Without Cre activity/induction | With Cre activity/induction |
Cre-positive, fl/△ | HET KO | HOM KO |
Cre-positive, fl/+ | WT | HET KO |
Cre-negative, fl/△ | HET KO | HET KO |
Cre-negative, fl/+ | WT | WT |
Summary of Basic Cre/loxP Breeding Guidelines
In conclusion, while Cre/loxP models are tremendously powerful due to their simple mechanism and widespread availability, the following best practices should always be followed:- All animals should always be screened for both the floxed and recombined alleles, using genotyping assays validated with both positive and negative controls.
- The floxed allele should always be maintained in a separate breeding colony (recommended) or cryopreserved stock (at minimum) and never exposed to Cre recombinase.
- Animals that do not inherit Cre but show unexpected recombination should not be used experimentally or for breeding, if possible, as this indicates unintended recombination in the germline or early embryo.
- Animals that inherit Cre but also show partial recombination at genotyping may result from either predictable and/or intended expression of Cre (e.g., within a biopsy sample) or unintended recombination. To guard against accidental propagation of the recombined allele, these animals should not be used for breeding unless necessary, and if necessary, should not be bred with one another.
- At each step in the breeding plan, one of the two parental genotypes placed into mating should be sourced from a separate breeding colony never exposed to Cre recombinase.
- Both the activity and expression of Cre, along with the potential for unintended recombination in the germline or early embryo, should always be tested empirically if possible. Further, it is ideal to directly test the floxed allele of interest (rather than a reporter allele).
- Always include the following controls for analysis alongside study cohorts, if possible: Cre-positive controls, Cre-negative controls, and wild-type controls. Based on the mating format(s) utilized, the exact genotype of such controls may vary and should be carefully considered.