Part II: Microbiome Workshop Topics
- Part I: Participants and Presentations
- Part II: Microbiome Workshop Topics
- Part III: Best Practices for Study Design
Mice in Microbiome Research
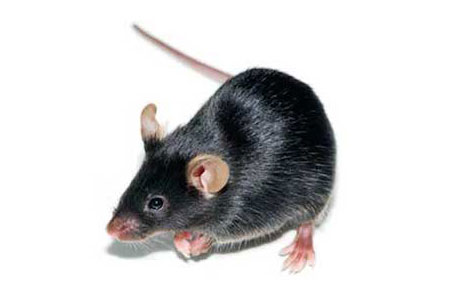
This is important from scientific, financial, and ethical perspectives, and the importance of combining mouse models with other approaches — such as in silico and in vitro modeling — was highlighted during the workshop. Presenters and participants repeatedly emphasized, however, that mouse models are vital to understanding mechanisms of action and for cause-effect demonstrations in a biologically relevant context.
There was a concern, shared by the presenters that the industry is moving too fast in the hunt for microbiome-based therapies. A deeper understanding of mechanism than we currently possess is necessary for a more targeted, evidence-based development of interventional opportunities.
The Gastrointestinal Tract and Microbiome of Mice and Humans
Mouse models have become more precise with the advent and continuous refinement of genetic engineering, but there are essential differences between mice and humans that need to be acknowledged. This is especially true with regards to the microbiome and the gastrointestinal tract.In 2015, Nguyen and coauthors reviewed the similarities and differences between mice and humans relevant to microbiome studies1. The overall anatomy of the gastrointestinal tract is quite comparable between mice and humans, with the most notable differences being the large cecum of mice functioning as a fermentation chamber, and differences in the topical distribution of Paneth and goblet cells.
Key differences between the resident microbiome of mice versus humans were discussed during the workshop, as also reviewed by Nguyen et al. Despite this disparity, it has repeatedly been reported that composition shifts and loss of diversity in the mouse microbiome is related to diseases such as diabetes, obesity, and colitis in a similar fashion as seen in humans, vindicating the predictive validity of mouse models.
Clinical Perspectives on Fecal Microbiota Transplantation
The need for reliable animal models to dose with specific bacteria of interest was identified at the workshop.It has been demonstrated in several studies that disease phenotypes may be transferred from human patients to mice via fecal microbiota transplantation (FMT). This concept is vital in demonstrating a causative role of the microbiome in development of disease. Moreover, when FMT can be used for replicating aspects of human diseases in mice, it opens the door for generating reproducible mouse models with improved construct and face validity.
Examples of FMT experiments in mice with interesting clinical perspectives are on the rise:
- Probably the most famous human-to-mouse phenotype transfer experiment was published in in 2013. Here, Ridaura et al. demonstrated that germ-free (GF) mice transplanted with feces from twins that were either obese or lean acquired the obese or lean phenotype according to their donors, though both were fed the same low fat diet2.
- A recent publication from 2017 demonstrated the transfer of traits of anxiety and diarrhea to mice via FMT from patients with irritable bowel syndrome and the co-morbidity anxiety3. Mice genetically susceptible to Parkinson's disease were shown to only develop disease in the presence of a microbiota and not under GF conditions, and likewise displayed mice with stool transplanted from Parkinson's patients more disease symptoms compared to mice that had stool from healthy people4.
FMT is additionally a potential treatment of disease, which can also be studied in mice. For instance, FMT in a mouse model of necrotizing enterocolitis (NEC) could alleviate features of NEC, underlining a promising therapeutic potential of this treatment in infants with NEC5.
Germ-free Mice: Gold Standard for FMT Studies
In a breakout group led by Philip Dubé and James Mutamba, participants debated whether germ-free (GF) mice or mice that have had their gut microbiota depleted with antibiotics are the best murine in vivo models for studying the microbiome.One argument in favor of generating pseudo-GF mice by antibiotic depletion is that GF mice lack microbiome-specific education of their immune system in early life. This is a significant concern in studies involving immunological parameters.
On the other hand, there are also important — and often ignored — caveats related to using antibiotic-treated mice as vehicles for FMT:
Mice with Human Microbiota
The breakout group led by Will DePaolo and Beth McCormick discussed considerations and pitfalls of generating mice with human microbiota by FMT.There are few reports demonstrating stability of a human microbiome in mice over generations, even under specific pathogen free (SPF) conditions11, and the lack of additional supportive data showing stability of a human microbiome over mouse generations was a concern of many of the workshop participants. Microbiota-humanized mice are applicable for short term hypothesis-driven studies, but there is a need to validate these models over the longer term.
Using diet to optimize FMT with human microbiota in mice was discussed at the workshop, particularly the optimal substrates for human-derived bacteria versus those of laboratory mice.
While no ideal methods were identified during the workshop, everyone agreed that studies exploring different strategies for optimal colonization and stability of mice with human microbiota are essential to move the field forward.
Another legitimate concern is the observation that mice with human microbiota can have immature immune systems12. Researchers should pursue studies identifying strategies to correct this, such as addition of certain microorganisms, diet modifications, or even genetic modification of the host and/or microbiota.
Microbiome Workshop Conclusions
One of the key messages delivered during the workshop was that the microbiome is something we should be thinking about no matter what we are studying. The resident microbiome of SPF mice can affect the performance of many disease models13,14, for example; there are many variables to observe when designing experiments and sourcing animal models of the microbiome.Check out Part III of this series for the workshop's conclusions on microbiome study design best practices, with a focus on in vivo mouse models.