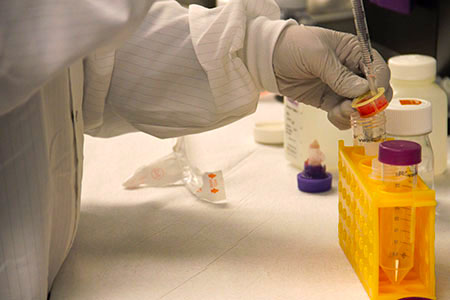
Applications of Rodent Irradiation
Irradiation preferentially kills rapidly dividing cells, including bone marrow and epithelial cells of the gut and other organs. The most common application of rodent irradiation is to destroy the bone marrow and other hematopoietic progenitors (myeloablation), either for immunosuppression or before replacing the immune system with donor grafts.
Sublethal radiation renders an animal transiently immunosuppressed; this is useful for engrafting tumors or hematopoietic stem cells. Such radiation doses are often used in conjunction with immunodeficient rodents to inhibit innate immune rejection, particularly by natural killer cells, or to enable the development of chimeric animals expressing several types of hematopoietic cells.
Complete myeloablation is accomplished by giving a lethal dose of radiation, generally followed by rescue of the immune system (i.e. preventing lethality) with bone marrow or hematopoietic stem cell transplantation. This makes it possible to replace the immune system of an animal with that of a donor, and to study how immune cells regulate transplant rejection, infection, autoimmunity, tumor growth, and more.
Irradiators for Rodent Research
These techniques are made possible by specialized research irradiators which deliver precise doses of radiation in a safe and controlled manner. Appropriate radiation dosage is the key to the successful irradiation of rodents — excess doses inevitably lead to morbidity, while insufficient doses hinder experimental success and interpretation.
Small animal research typically employs one of two types of irradiators, gamma or X-ray.
- Self-shielded gamma irradiators use radioactive sources (137Cs or 60Co) that emit gamma radiation at discrete energies due to nuclear decay (0.662 MeV for 137Cs and 1.173/1.332 MeV for 60Co). Gamma irradiators are reliable radiation sources, but come with responsibility for radioisotope security and the ultimate disposal of spent sources.
- X-ray irradiators use electricity to power X-ray tubes to radiate over a wider spectrum of energy compared to that of gamma irradiators. X-ray irradiators do not require radioactive material and can be easily turned on or off. Possible limitations of X-ray irradiators include the spectrum of beam energies and the cost of X-ray tube maintenance.
Gamma vs. X-ray Irradiation
Radiation dosage should not be confused with its relative biological effect (RBE), which is a function of the beam's characteristics and the tissues with which it interacts. Different tissues within the body respond differently to the same radiation dose, depending on cellular characteristics, tissue composition, and depth, and these interactions can differ between various sources of radiation.
RBE cannot be accurately predicted and should be validated for different irradiator sources and experimental goals.
Both gamma and X-ray sources are effective for myeloablation and are often considered equivalent; however, caution should be taken in validation and replication of studies between these sources as they may induce different biological effects.
In general, the RBE for X-rays is higher than for that of gamma radiation by as much as 30-40%1, meaning that a lower dose (measured in Gy) may be required for X-ray than gamma irradiation. The use of filters to reduce low energy X-rays mitigates some of this difference, but variable effects on specific cell types2 and reconstitution efficiency3 have been reported between these two modalities.
These data suggest that model performance and off-target effects may be different between gamma and X-ray sources, and that validation and dose adjustment may be necessary.
Dosage Considerations
Radiation dose is usually denoted in terms of the SI unit gray (Gy or cGy) or the rad, where 1 rad equals 0.01 Gy; these terms are often interchangeable in animal research and dosages are indicated as either Gy, cGy or rad.
Care should be taken when interconverting between these units, so as to not deliver an inappropriate dose.
Units:
Absorbed radiation: 1 Gy (gray, SI unit) = 100 cGy = 100 radDose rate: Gy/min
Absorbed dose should not be confused with biological effect measured in Sv (sievert, SI unit) or rem (roentgen equivalent man).
Dosage Rates
Another key factor for rodent irradiation is the dose rate of the irradiator, typically indicated in Gy/min. This determines the length of time subjects should be exposed to a beam for a desired radiation dosage.
Many factors determine the dose rate of an irradiator. Failure to take these into account will lead to incorrect calculation of exposure times and dosing4.
- Source activity
- X-ray beam energy
- Position/distance
- Stationary vs. rotating subjects
- Presence of attenuators/filters
Accurate calibration and proper usage of an irradiator is essential. Users are encouraged to seek advice if unsure.
In the case of 137Cs or 60Co irradiators, the radioactive decay of these isotopes predictably increases the irradiation time required based on isotope half-lives of 30.17 and 5.27 years, respectively. With 137Cs irradiators, for example, the exposure time required will increase two-fold over 30 years, or approximately 2.3% per year.
Typical Radiation Doses and Considerations
Dosage is determined by several factors, which may impact the performance of your model. These should be considered when validating new protocols:
- Radiation source: X-rays may require lower dosages than gamma irradiation. The power output of the X-ray tube and the presence of shielding may affect this.
- Strain background: BALB/c and scid mice require lower dosages.
- Age: Dosages may need to be decreased for periods of sensitivity or increased for periods of resistance during aging5. Of note, there are large changes in radiosensitivity between 1 and 3 months of age:
- Neonatal: relatively resistant
- Weaning (20-30 days): most sensitive
- Young adulthood (3-4 months): most resistant
- Aged (6 months +): declining resistance throughout age
- Health status: Health status and body weight/condition: Health issues may lead to lower tolerated doses (e.g. pathogens, opportunists, stress, malnutrition and underlying disease).
- Time of day: There are diurnal variations in radiation sensitivity6.
- Multiple doses: Fractionating a dose by delivering 2 half doses separated by 3-4 h has been shown to reduce radiation complications with equivalent myeloablation in C57BL/6 mice7.
Mouse Strain-Dependent Effects of Radiation
There are well established differences in the radiosensitivity of different inbred mouse strains8-14, which should be considered in models requiring irradiation.
- BALB/c mice are particularly sensitive to the effects of radiation due to an unknown autosomal recessive genetic locus10,11, and cannot tolerate the same radiation dose as C57BL/6 mice.
- F1 hybrids from C57BL/6 x BALB/c crosses are similarly radioresistant to parental C57BL/6; however, crossing these hybrids further reveals a range of different radiosensitivities in F2 and F3 generations.
Genetic variations in radiation injury may be problematic when using mice on mixed inbred backgrounds, such as transgenic or genetically modified mice, leading to unpredictable responses to radiation within research cohorts. Working with mice on defined genetic backgrounds is advisable for irradiation studies.
Mice with defective DNA repair pathways are most highly-susceptible to radiation-induced injury. A prime example of this are scid mice, where a mutation in Prkdc leads to defective double-strand break DNA repair. Any mouse with the Prkdcscid mutation will be extremely sensitive to radiation and should receive greatly reduced dosages compared to other mouse strains14; this includes: C.B-17 scid, ICR scid, NOD scid, scid-beige, and NOG.
129S ≤ SJL ≤ C3H ≤ C57BL/6 << BALB/c << scid
Typical Strain Dosage Ranges
Typical dose (cGy) | |
C57BL/6 | Complete myeloablation (lethal dose): Single dose: 900-1100 Fractionated dose: 600 + 600 with 3-4 h interval Partial myeloablation (sublethal dose):Single dose of 350-600 |
BALB/c | Complete myeloablation (lethal dose): Single dose: 700-800 maximum Partial myeloablation (sublethal dose):Single dose: 600 or less |
scid | Immunosuppression: 50-250 |
Note: 1 Gy = 100 cGy = 100 rad
Health Standards and Rodent Irradiation
Irradiation typically renders mice immunosuppressed; depending on dosage, this effect may be transient (lasting until hematopoietic cells are replenished through endogenous recovery or transplant) or permanent.
During this period, mice are susceptible to opportunistic infections that may affect survival or model performance. Of particular importance is Pseudomonas aeruginosa, which can translocate through the gut in irradiated animals, leading to bacteremia and sepsis15.
Selecting animals with appropriate health standards, or using prophylactic antibiotic therapy and aseptic techniques, can prevent this and other opportunistic complications following irradiation.
Rodent Irradiation Best Practices
- Ensure that irradiators have been properly calibrated and that users are trained.
- Validate specific sources of radiation and dosage rates for biological responses and model performance.
- Consider specific biological variables (strain, age, health status) and adjust radiation dosages for optimal responses and acceptable side effects.
- Report the type of irradiator used, the total dose administered, and the dosage rate in studies.
- Report the age and health status of animals at the time of irradiation.
- Consult with your institutional veterinarian for information into the performance of available irradiators for specific studies.